Catalysts design and mechanistic studies
Multielectron/multiproton transfer reactions are at the core of important biological and chemical processes, such as small molecule activation and energy conversion. Optimization of these kinetically constrained reactions is a critical challenge for energy-related applications. Nature widely utilizes first-row transition metals in enzymes to catalyze these processes under ambient conditions and we develop a bioinspired approach to design bioinspired catalytic platforms for H2 evolution, CO2 valorization and N2 fixation.
We further exploit all these catalysts through integration in carbon nanotube-based electrodes (Axis II) or assembly with various light-harvesting units to form artificial photosynthetic systems (Axis III).
H2 evolution
In particular, hydrogen production and uptake are two reactions efficiently catalyzed by enzymatic sites such as the dinuclear NiFe and FeFe clusters in hydrogenases and during the last decade, intensive research efforts have been devoted in our team to the development of biomimetic and bioinspired H2-evolving catalysts based on Ni, Fe and Co centres.
The design of biomimetic catalysts for H2 evolution has been the core expertise of the SolHyCat team since 2002. Since 2006, our group has reported novel catalysts reproducing key structural, spectroscopic and reactivity features of the active site of [NiFe] hydrogenases.
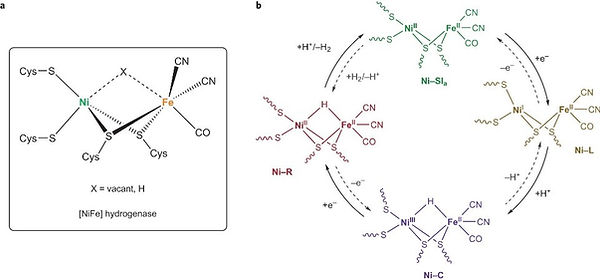
We also investigate cobalt complexes as H2 evolution catalysts. This includes our earlier work on cobaloximes and cobalt diimine-dioxime complexes as well as our current development of novel catalytic platforms such as the [Co(bapbpy]2+ complexes. For all these molecular catalysts, we apply state-of-the art analysis of the electrocatalytic response to determine the catalytic mechanism, identify the role of redox-active moieties and proton relays (oxime bridge, pendant amine groups…) and benchmark their catalytic activity with other molecular catalysts for the literature.


CO2 valorization and N2 fixation
The active sites of CODH and formate-dehydrogenase contain basic sites in the vicinity of the metal sites where CO2 is activated. We apply bio-inspiration concepts to design novel molecular catalytic platforms for CO2 conversion. In that prospect, cobalt complexes such as [CpCoP2N2)] (figure) again prove quite promising.
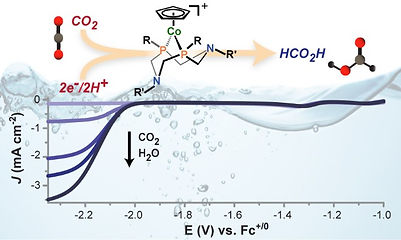
A natural evolution of our research interests to even more challenging multielectron/multiproton transfer reactions led us to question the fundamental basis of nitrogen reduction to ammonia by diazotrophic organisms. To this purpose, we are actively developing versatile multivalent ligands platforms able to stabilize discrete metal sulfide clusters reminiscent of the natural poly(hetero)metallic sulfides co-factors that are supporting nitrogen reduction in nitrogenases. A systematic investigation of the reactivity of our synthetic constructs towards various substrates of this enzyme will help us deciphering the critical parameters governing the activity of this fascinating class of biomolecules
Integrating bio-inspired catalysts into Electrolysers and Fuel Cells
Grafting molecular catalysts for Hydrogen production/uptake on nanostructured electrodes
Incorporating molecular catalysts in devices for hydrogen production/uptake is a major goal in the perspective of their implementation into technological devices such as fuel-cells and electrolyzers. As stated in the introduction, the covalent grafting onto an electrode material such as carbon or the incorporation of catalyst into a polymer coating an electrode would lead to new electrocatalytic materials for hydrogen production or uptake. We have developed an original strategy, combining the biomimetic molecular approach developed in Axis I with nanochemical tools and shown that the covalent attachment of a nickel-bisdiphosphine mimic of the active site of hydrogenase enzymes on carbon nanotubes results in a nickel-based cathode nanomaterial (Figure 1) with remarkable performances under the strongly acidic conditions required in the expanding proton exchange membrane (PEM) technology.
These materials are unique catalysts for hydrogen oxidation under highly acidic conditions with current densities reaching >20 mA/cm2 even in the presence of carbon monoxide (CO), a major impurity in H2 fuels derived from reformed hydrocarbons or biomass. This constitutes a major breakthrough for Nafion-based PEM fuel cells technology since CO poisoning limits the commercialization of devices based on Pt electrocatalysts. We demonstrated their possible integration in noble metal-free PEMFC fuel cell. Our program combines formulation studies as well as detailed physico-chemical characterization to progress towards educated and rational optimization of their performances with the objective of reaching current densities compatible with technological integration.

The same methodologies can be applied to elaborate molecular–engineered H2 evolution catalytic materials. Grafting indeed provides a largely increased protection of these catalysts against degradation. some of these immobilized catalysts prove tolerant to the presence of oxygen in the media.
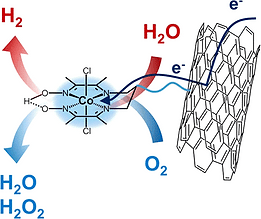
Bio-inspired and electrodeposited solid-state catalytic materials
As an alternative approach to the grafting of molecular catalysts onto a suitable electrode substrate, we also investigate the preparation of novel solid-state electrocatalytic materials. Our program specifically targets metal sulfides that mimic some of the catalytic properties of the active sites of metalloenzymes such as hydrogenases, CO-dehydrogenases or nitrogenases. Such metal sulfide materials prove quite active as H2 evolution catalysts from acidic solutions and we formulate them in catalytic layers of proton-exchange membrane electrolyzers.
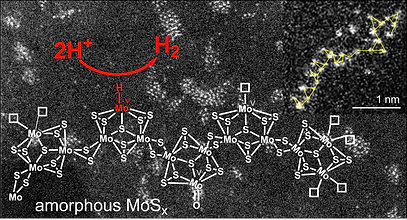